2,3-Dihydroxybenzoic acidCAS# 303-38-8 |
Quality Control & MSDS
Number of papers citing our products
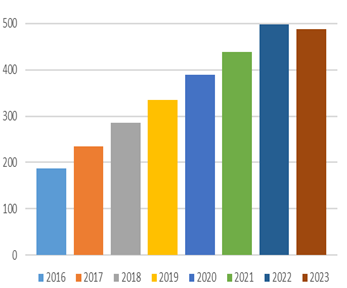
Chemical structure
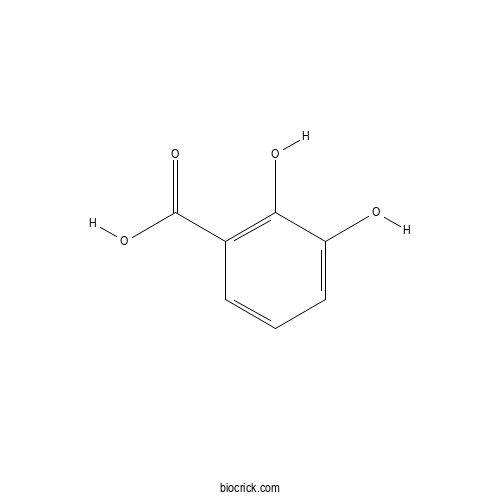
3D structure
Cas No. | 303-38-8 | SDF | Download SDF |
PubChem ID | 19 | Appearance | Powder |
Formula | C7H6O4 | M.Wt | 154.1 |
Type of Compound | Phenols | Storage | Desiccate at -20°C |
Solubility | Soluble in Chloroform,Dichloromethane,Ethyl Acetate,DMSO,Acetone,etc. | ||
Chemical Name | 2,3-dihydroxybenzoic acid | ||
SMILES | C1=CC(=C(C(=C1)O)O)C(=O)O | ||
Standard InChIKey | GLDQAMYCGOIJDV-UHFFFAOYSA-N | ||
Standard InChI | InChI=1S/C7H6O4/c8-5-3-1-2-4(6(5)9)7(10)11/h1-3,8-9H,(H,10,11) | ||
General tips | For obtaining a higher solubility , please warm the tube at 37 ℃ and shake it in the ultrasonic bath for a while.Stock solution can be stored below -20℃ for several months. We recommend that you prepare and use the solution on the same day. However, if the test schedule requires, the stock solutions can be prepared in advance, and the stock solution must be sealed and stored below -20℃. In general, the stock solution can be kept for several months. Before use, we recommend that you leave the vial at room temperature for at least an hour before opening it. |
||
About Packaging | 1. The packaging of the product may be reversed during transportation, cause the high purity compounds to adhere to the neck or cap of the vial.Take the vail out of its packaging and shake gently until the compounds fall to the bottom of the vial. 2. For liquid products, please centrifuge at 500xg to gather the liquid to the bottom of the vial. 3. Try to avoid loss or contamination during the experiment. |
||
Shipping Condition | Packaging according to customer requirements(5mg, 10mg, 20mg and more). Ship via FedEx, DHL, UPS, EMS or other couriers with RT, or blue ice upon request. |
Description | 2,3-Dihydroxybenzoic acid, a iron chelator, is a new growth factor for multiple aromatic auxotrophs. 2,3-Dihydroxybenzoic acid attenuates kanamycin-induced volume reduction in mouse utricular type I hair cells. It can effectively interfere with the deleterious effects of H2O2 in monocytes. |

2,3-Dihydroxybenzoic acid Dilution Calculator

2,3-Dihydroxybenzoic acid Molarity Calculator
1 mg | 5 mg | 10 mg | 20 mg | 25 mg | |
1 mM | 6.4893 mL | 32.4465 mL | 64.8929 mL | 129.7859 mL | 162.2323 mL |
5 mM | 1.2979 mL | 6.4893 mL | 12.9786 mL | 25.9572 mL | 32.4465 mL |
10 mM | 0.6489 mL | 3.2446 mL | 6.4893 mL | 12.9786 mL | 16.2232 mL |
50 mM | 0.1298 mL | 0.6489 mL | 1.2979 mL | 2.5957 mL | 3.2446 mL |
100 mM | 0.0649 mL | 0.3245 mL | 0.6489 mL | 1.2979 mL | 1.6223 mL |
* Note: If you are in the process of experiment, it's necessary to make the dilution ratios of the samples. The dilution data above is only for reference. Normally, it's can get a better solubility within lower of Concentrations. |
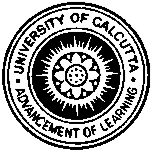
Calcutta University
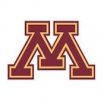
University of Minnesota
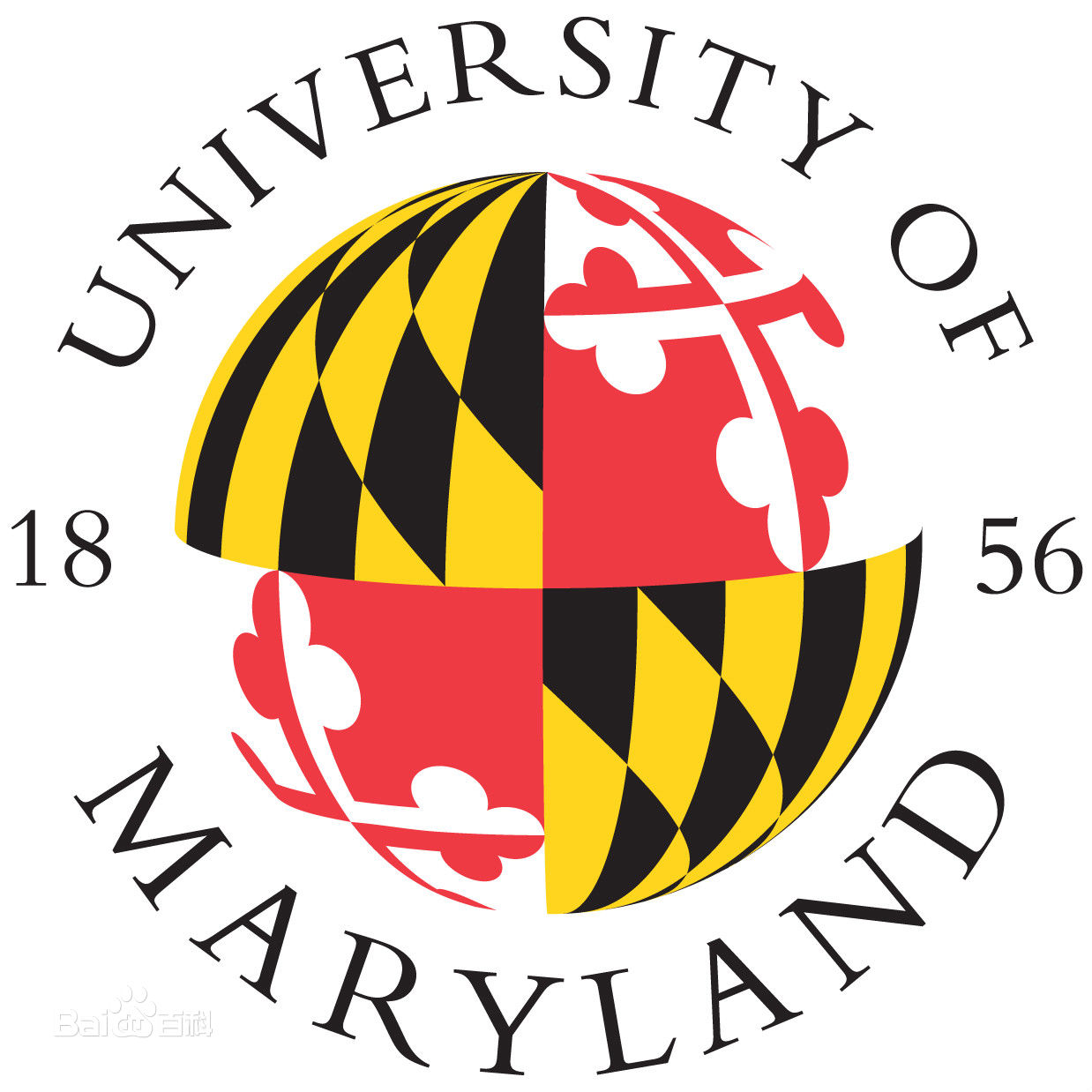
University of Maryland School of Medicine
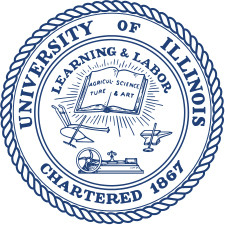
University of Illinois at Chicago
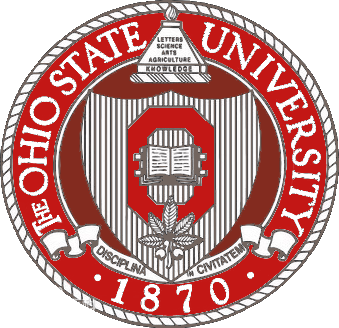
The Ohio State University
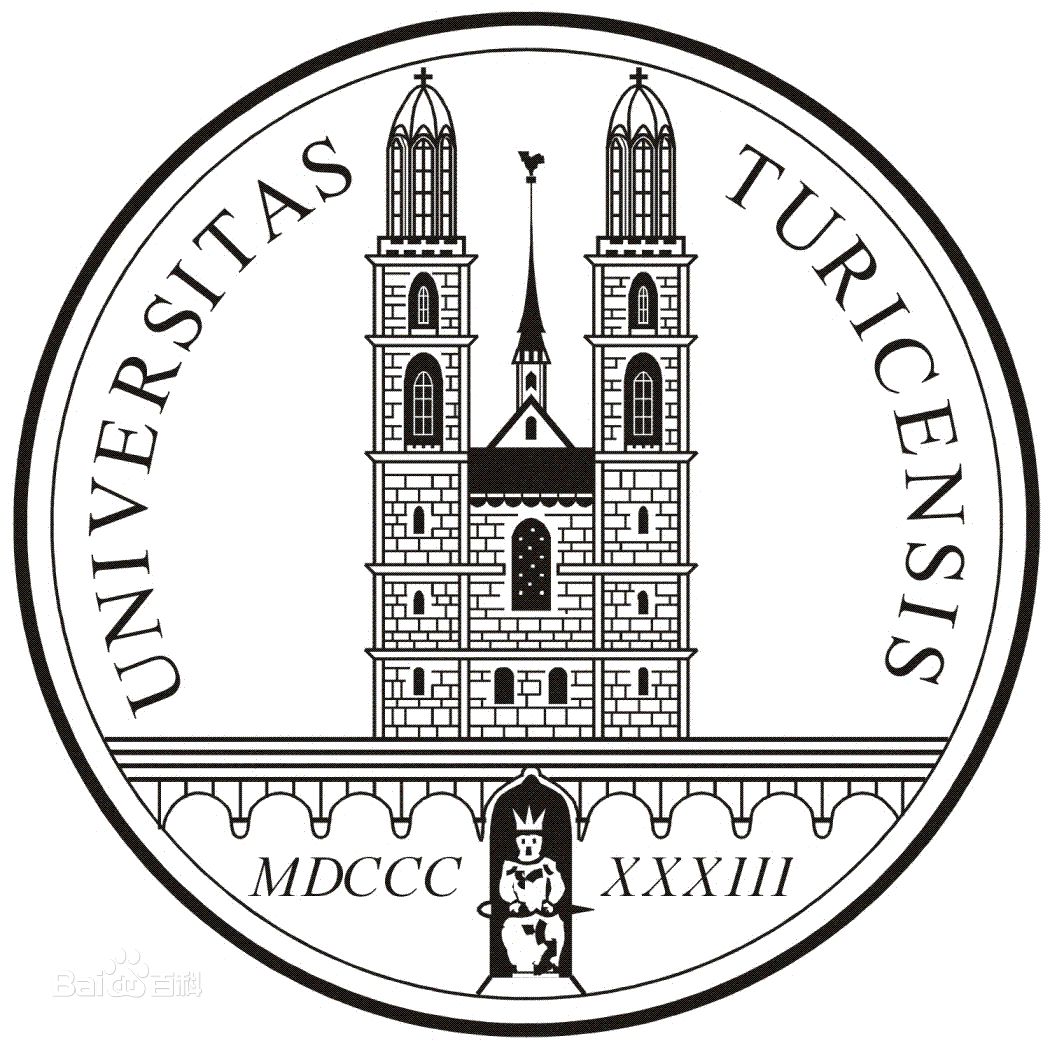
University of Zurich
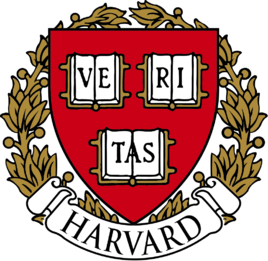
Harvard University
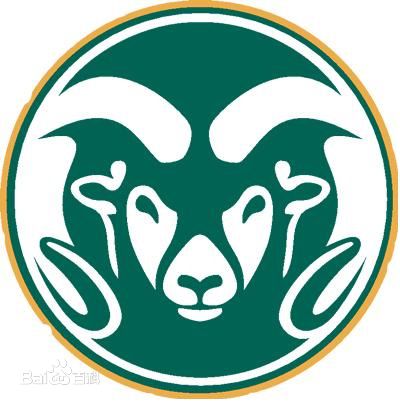
Colorado State University
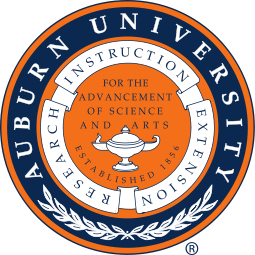
Auburn University
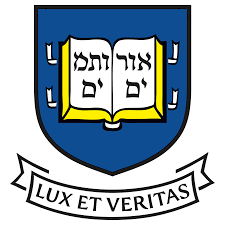
Yale University
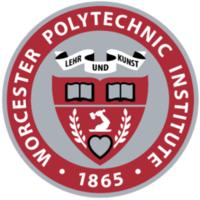
Worcester Polytechnic Institute
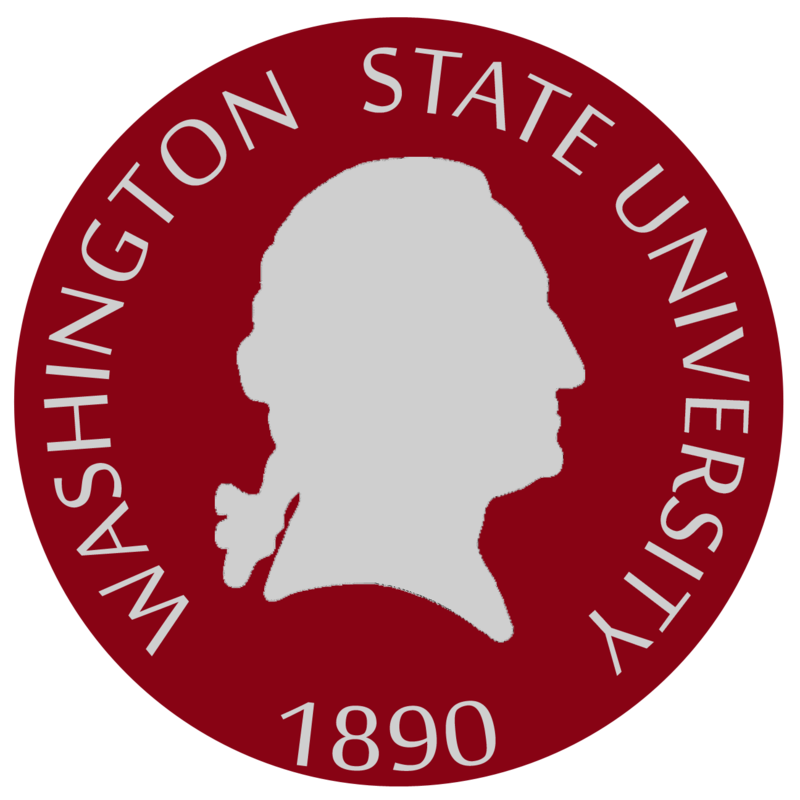
Washington State University
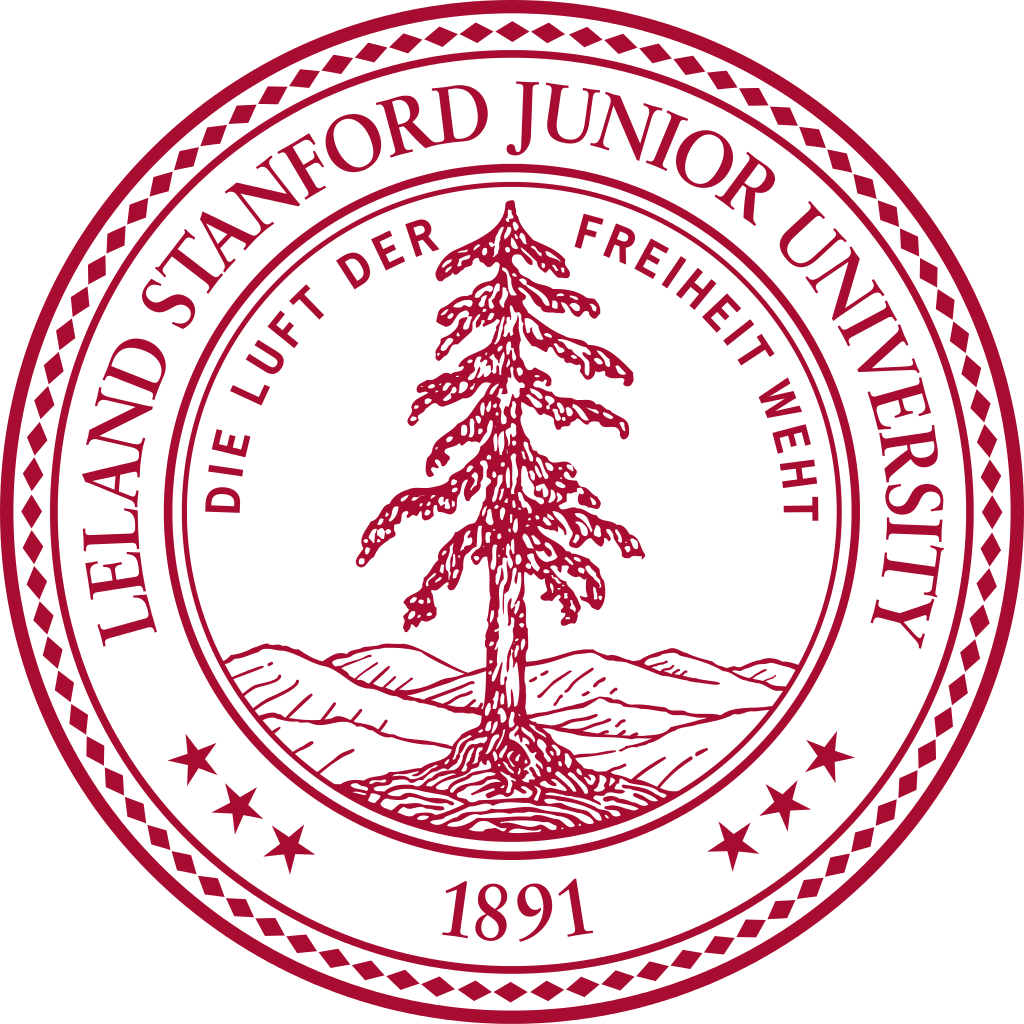
Stanford University
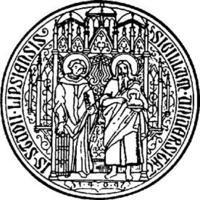
University of Leipzig
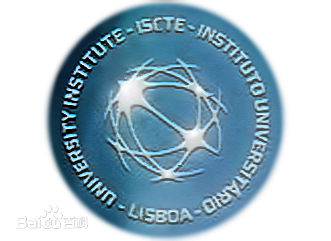
Universidade da Beira Interior
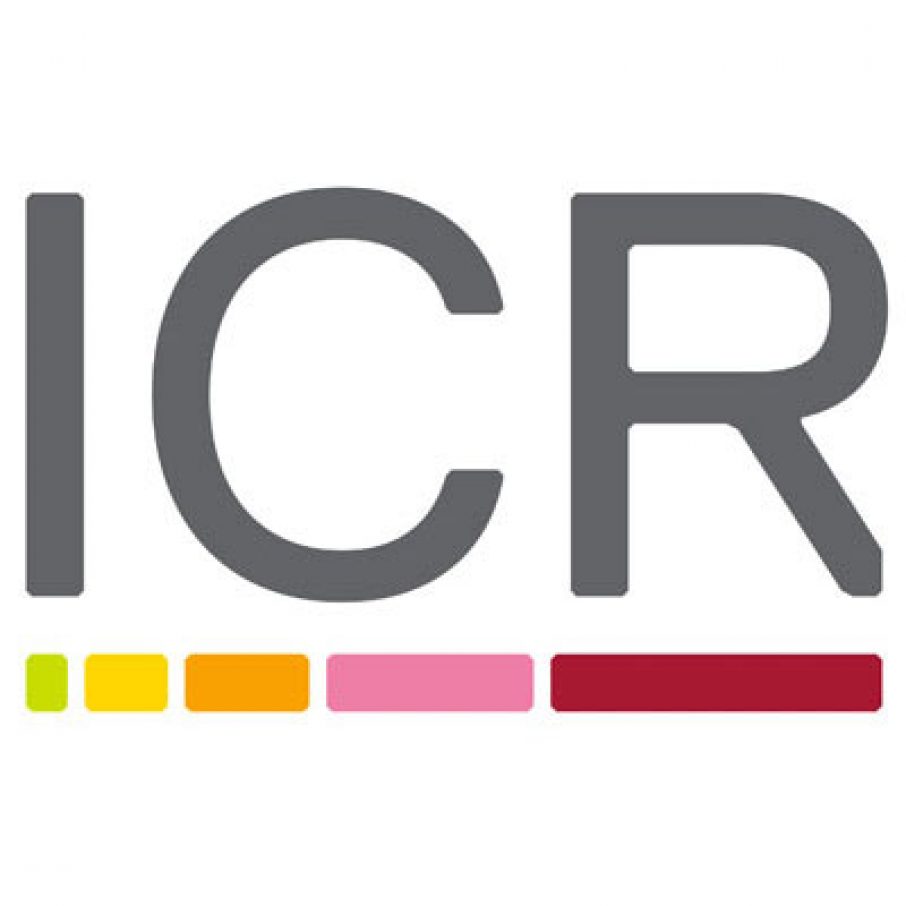
The Institute of Cancer Research
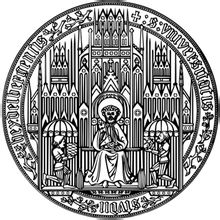
Heidelberg University
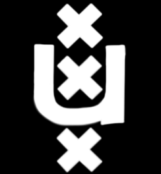
University of Amsterdam
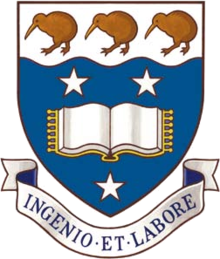
University of Auckland
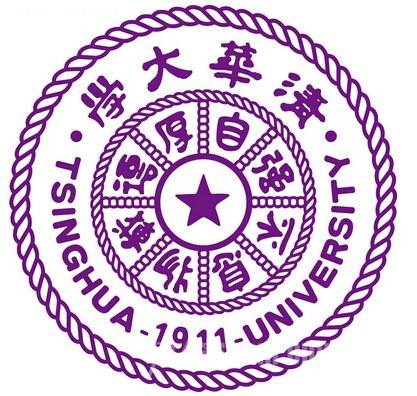
TsingHua University
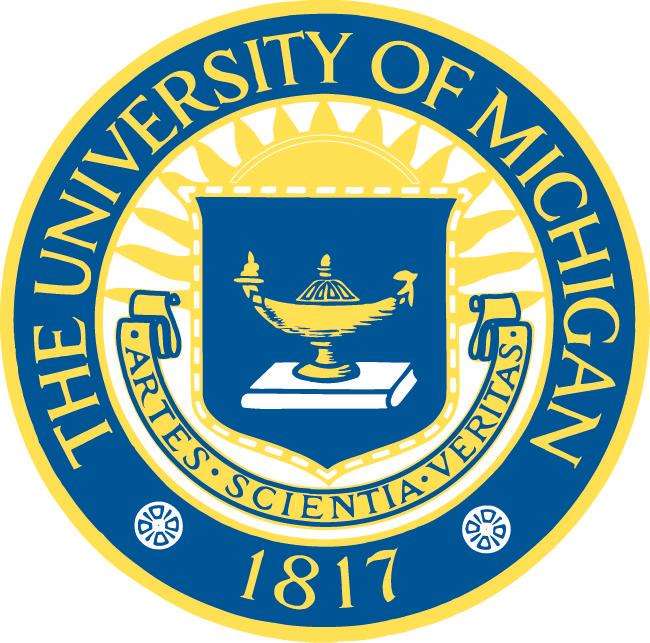
The University of Michigan
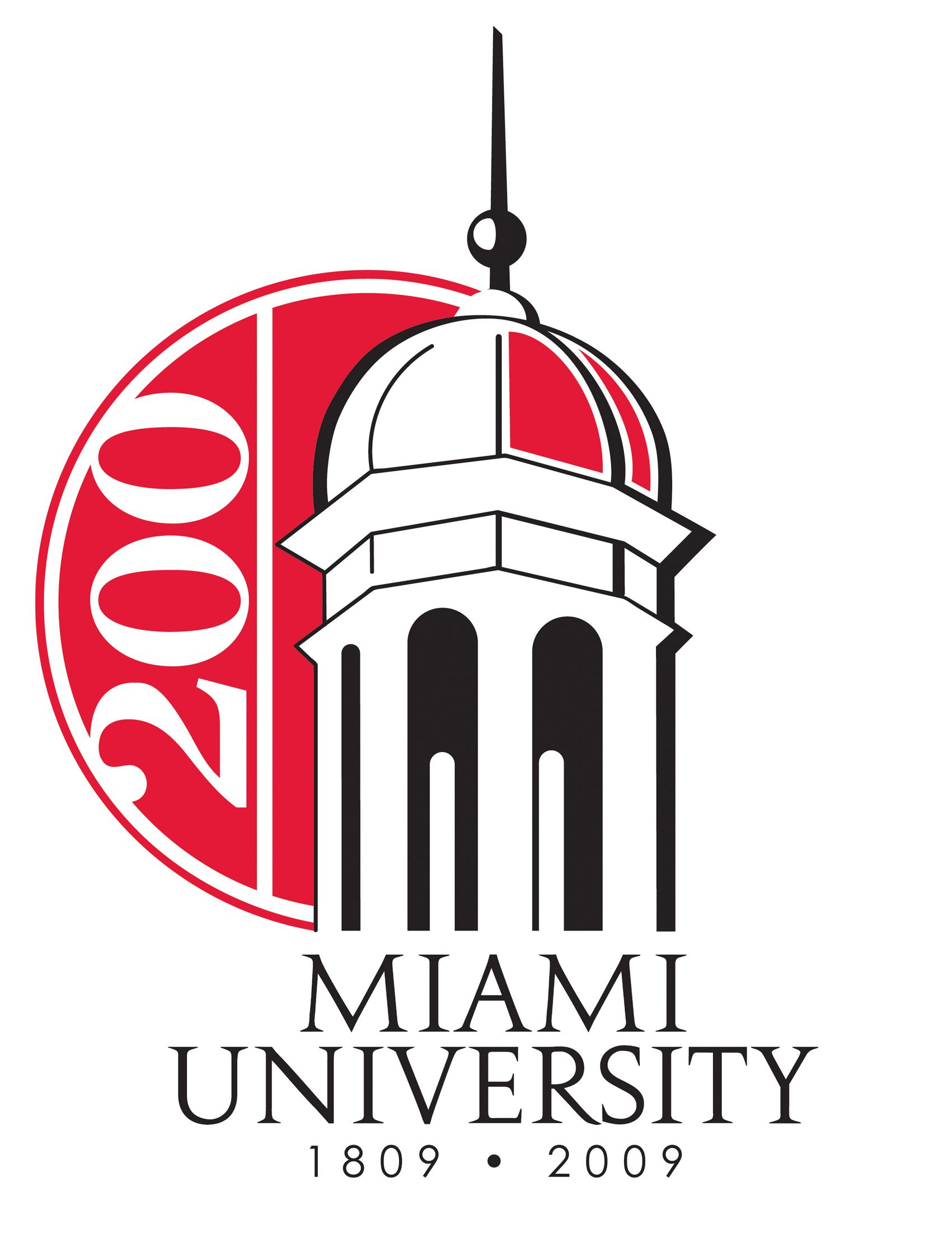
Miami University
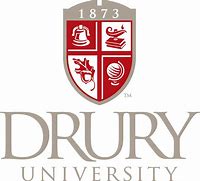
DRURY University
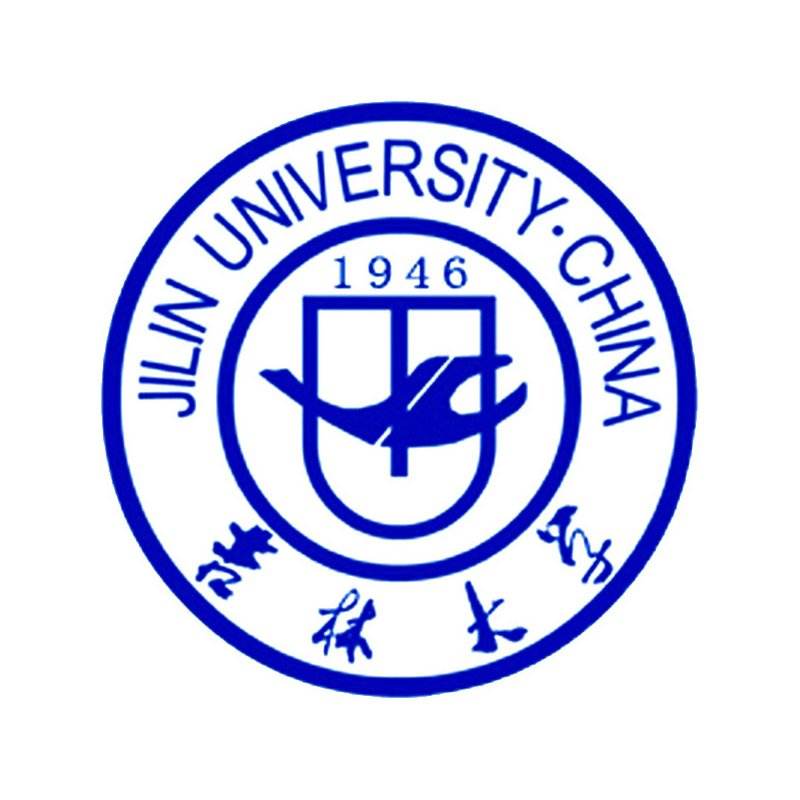
Jilin University
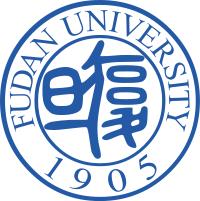
Fudan University
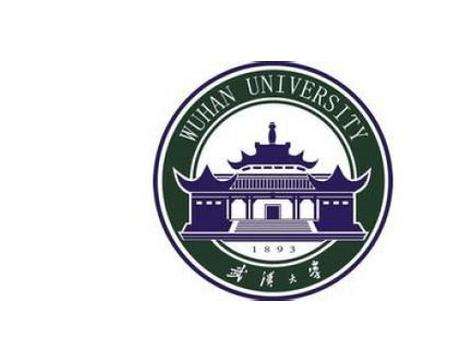
Wuhan University
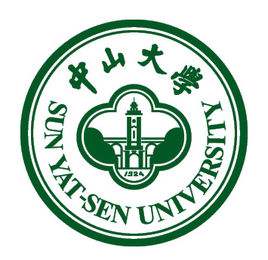
Sun Yat-sen University
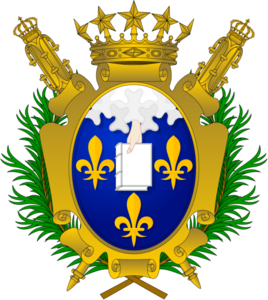
Universite de Paris
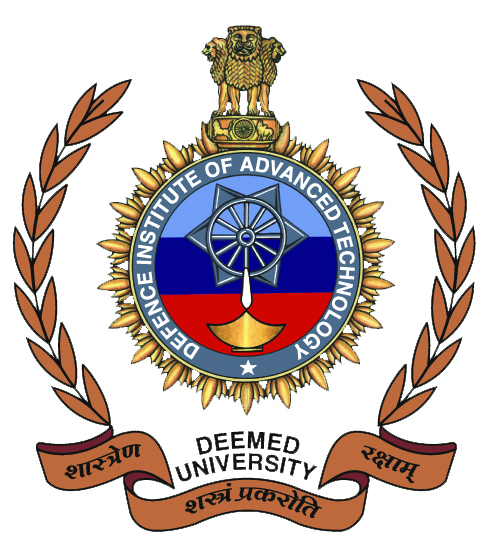
Deemed University
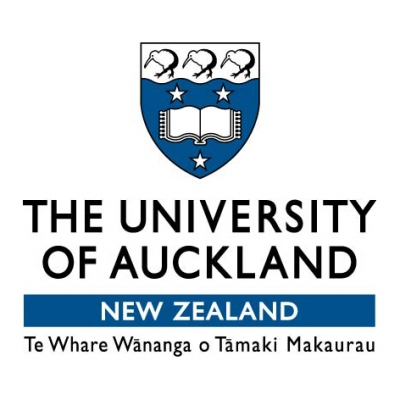
Auckland University
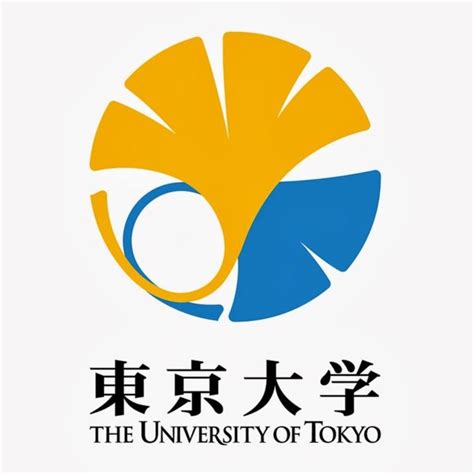
The University of Tokyo
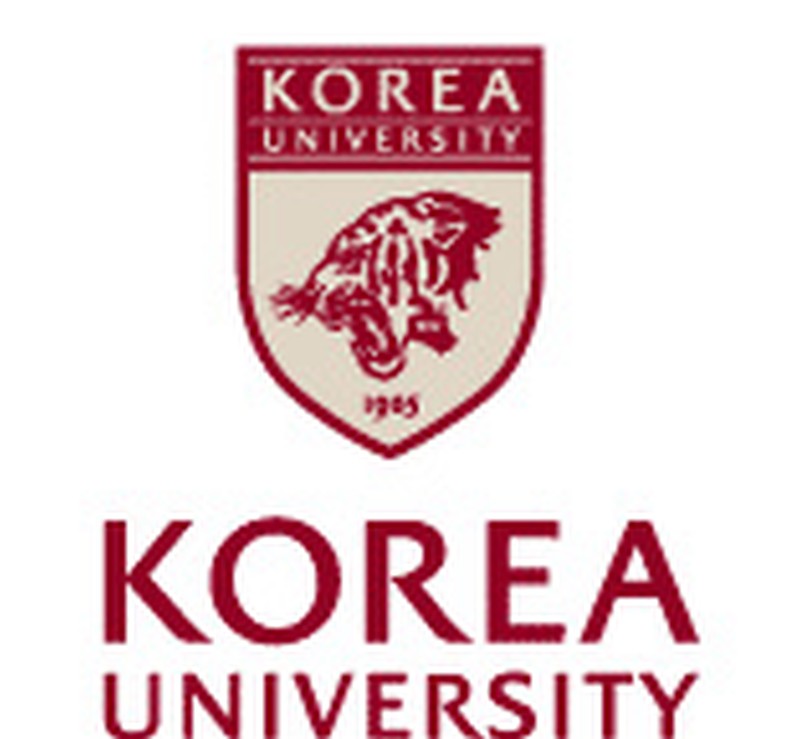
Korea University
- Ethyl isovalerate
Catalog No.:BCN0166
CAS No.:108-64-5
- Yohimbic acid monohydrate
Catalog No.:BCN0165
CAS No.:522-87-2
- 5-Hydroxyflavone
Catalog No.:BCN0164
CAS No.:491-78-1
- 7-Hydroxy-4'-methoxyflavone
Catalog No.:BCN0163
CAS No.:487-24-1
- Qingdainone
Catalog No.:BCN0162
CAS No.:97457-31-3
- 2,3-Dimethylanthraquinone
Catalog No.:BCN0161
CAS No.:6531-35-7
- 2,5-Dimethoxybenzoic acid
Catalog No.:BCN0160
CAS No.:2785-98-0
- Bicyclo[5.3.0]decapentaene
Catalog No.:BCN0159
CAS No.:275-51-4
- (E)-N-(2-Amino-4-fluorophenyl)-3-(1-cinnamyl-1H-pyrazol-4-yl)acrylamide
Catalog No.:BCN0158
CAS No.:1396841-57-8
- 2-Hydroxy-3-methoxybenzaldehyde
Catalog No.:BCN0157
CAS No.:148-53-8
- Lappaol B
Catalog No.:BCN0156
CAS No.:62359-60-8
- N-trans-caffeoyltyramine
Catalog No.:BCN0155
CAS No.:103188-48-3
- trans-Chrysanthemyl alcohol
Catalog No.:BCN9908
CAS No.:5617-92-5
- 6-Hydroxy-7-methoxydihydroligustilide
Catalog No.:BCN0168
CAS No.:210036-09-2
- Perlolyrine
Catalog No.:BCN0169
CAS No.:29700-20-7
- 3-Oxo-4-benzyl-3,4-dihydro-1H-pyrrolo [2,1-c] oxazine-6-methylal
Catalog No.:BCN0170
CAS No.:60026-28-0
- Eriodictyol 7-O-glucuronide
Catalog No.:BCN0171
CAS No.:125535-06-0
- Corymboside
Catalog No.:BCN0172
CAS No.:73543-87-0
- Securoside A
Catalog No.:BCN0173
CAS No.:107172-40-7
- Isosaponarin 2''-O-glucoside (Isovitexin-2''-4'-di-O-beta-D-glucoside)
Catalog No.:BCN0174
CAS No.:63316-27-8
- Sibiricose A4
Catalog No.:BCN0175
CAS No.:241125-73-5
- Glaucoside C
Catalog No.:BCN0176
CAS No.:81474-89-7
- Margaritene
Catalog No.:BCN0177
CAS No.:64271-10-9
- 11-Oxomogroside IIA2
Catalog No.:BCN0178
CAS No.:2170761-37-0
Metal Ion Promiscuity and Structure of 2,3-Dihydroxybenzoic Acid Decarboxylase of Aspergillus oryzae.[Pubmed:33090643]
Chembiochem. 2020 Oct 14.
Broad substrate tolerance and excellent regioselectivity, as well as independence from sensitive cofactors established benzoic acid decarboxylases from microbial sources as efficient biocatalysts. Robustness under process conditions makes them particularly attractive for preparative-scale applications. The divalent metal-dependent enzymes are capable of catalyzing the reversible non-oxidative (de)carboxylation of a variety of electron-rich (hetero)aromatic substrates analogously to the chemical Kolbe-Schmitt reaction. Elemental mass spectrometry supported by crystal structure elucidation and quantum chemical calculations verified the presence of a catalytically relevant Mg 2+ complexed in the active site of 2,3-dihydroxybenoic acid decarboxylase from Aspergillus oryzae (2,3-DHBD_ Ao ). This unique example with respect to the nature of the metal is in contrast to mechanistically related decarboxylases, which generally bear Zn 2+ or Mn 2+ as the catalytically active metal.
(-)-Epicatechin and the colonic metabolite 2,3-dihydroxybenzoic acid protect against high glucose and lipopolysaccharide-induced inflammation in renal proximal tubular cells through NOX-4/p38 signalling.[Pubmed:32959859]
Food Funct. 2020 Oct 21;11(10):8811-8824.
Chronic hyperglycaemia and inflammation are present in diabetes and both processes have been related to the pathogenesis of diabetic kidney disease. Epicatechin (EC) and main colonic phenolic acids derived from flavonoid intake, such as 2,3-Dihydroxybenzoic acid (DHBA), 3,4-dihydroxyphenylacetic acid (DHPAA) and 3-hydroxyphenylpropionic acid (HPPA), have been suggested to exert beneficial effects in diabetes. This study was aimed at investigating whether the mentioned compounds could prevent inflammation in renal proximal tubular NRK-52E cells induced by high glucose and lipopolysaccharide (LPS). Pre-treatment of cells with EC and DHBA (5 muM) reverted the enhanced levels of pro-inflammatory cytokines, such as tumour necrosis factor-alpha (TNF-alpha), interleukin-6 (IL-6) and monocyte chemoattractant protein 1 (MCP-1), activated by high glucose and LPS. Additionally, EC and DHBA pre-incubation reduced the increased values of adhesion molecules, namely, intercellular cell adhesion molecule-1 (ICAM-1) and vascular cell adhesion molecule-1 (VCAM-1), as well as those of mitogen-activated protein kinases (MAPKs) [extracellular signal-regulated kinase (ERK), -c-jun N-terminal kinase (JNK) and -p38 protein kinase (p38)] activated by the high glucose and LPS challenge. Thus, in EC and DHBA pre-treated cells ICAM-1, p-ERK and p-JNK were returned to control values, and VCAM-1 and p-p38 levels were reduced by approximately 20 and 25%, respectively, when compared to high glucose plus LPS-stimulated cells. Likewise, pre-treatment with EC and DHBA protected against high glucose plus LPS-triggered oxidative stress by preventing increased ROS and NADPH oxidase 4 (NOX-4) levels ( approximately 25 and 45% reduction, respectively). By using specific inhibitors of p38 and NOX-4, the participation of both proteins in EC- and DHBA-mediated protection against inflammation and associated oxidative stress was shown. Taken together, EC and DHBA exert beneficial effects in renal proximal tubular cells, as they contribute to preventing the inflammatory-induced milieu and the accompanying redox imbalance, playing NOX-4/p38 a crucial role.
Precursor-directed biosynthesis of catechol compounds in Acinetobacter bouvetii DSM 14964.[Pubmed:32926028]
Chem Commun (Camb). 2020 Oct 13;56(81):12222-12225.
Genome mining for VibH homologs reveals several species of Acinetobacter with a gene cluster that putatively encodes the biosynthesis of catechol siderophores with an amine core. A. bouvetii DSM 14964 produces three novel biscatechol siderophores: propanochelin (1), butanochelin (2), and pentanochelin (3). This strain has a relaxed specificity for the amine substrate, allowing for the biosynthesis of a variety of non-natural siderophore analogs by precursor directed biosynthesis. Of potential synthetic utility, A. bouvetii DSM 14964 condenses 2,3-Dihydroxybenzoic acid (2,3-DHB) to allylamine and propargylamine, producing catecholic compounds which bind iron(iii) and may be further modified via thiol-ene or azide-alkyne click chemistry.
Stepwise Coordination Assembly Approach toward Aluminum-Lanthanide-based Compounds.[Pubmed:32883079]
Inorg Chem. 2020 Sep 21;59(18):13760-13766.
Present herein is a stepwise assembly method toward aluminum-lanthanide-based (Al-Ln) compounds. From the perspective of charge balance, polyanions are necessary to bind with Ln ions. However, the synthesis of polyanions aluminum compounds remains quite challenging. Herein, two Al4 polyanions [Al4(L)4(Cat)2].4Hdma (AlOC-13, H3L= 2,3-Dihydroxybenzoic acid, Cat = catechol, and dma = dimethylamine) and [Al4(L)4(HL)2(DMF)2].4Hdma.0.5DMF.0.5H2O (AlOC-14, DMF = N,N-dimethylformamide) were successfully obtained under solvothermal conditions. Catechol and Hdma were generated from the in situ decarboxylation of H3L ligand and decomposition of DMF, respectively. AlOC-13 is qualified for further coordination assembly for the available vacancy coordination sites, good water solubility, and scale-up synthesis. The assembly of Al4 polyanions with equivalent Ln ions afforded a series of zigzag chain structures [LnAl4(L)4(Cat)2(DMF)2(H2O)3].Hdma (Ln = Sm, Eu, Gd, Tb, Dy, Ho, Er, Yb) (AlOC-13-Ln). Moreover, the magnetic behavior and photoluminescence of the series of AlOC-13-Ln were also studied. AlOC-13-Dy shows obvious antiferromagnetic behavior, while AlOC-13-Tb exhibits excellent green characteristic luminescence. This study not only paves the way toward anionic aluminum clusters but also reveals their potential application in water treatment of cationic metal ions capture.
Antioxidant Activity of Selected Phenolic Acids-Ferric Reducing Antioxidant Power Assay and QSAR Analysis of the Structural Features.[Pubmed:32645868]
Molecules. 2020 Jul 7;25(13). pii: molecules25133088.
Phenolic acids are naturally occurring compounds that are known for their antioxidant and antiradical activity. We present experimental and theoretical studies on the antioxidant potential of the set of 22 phenolic acids with different models of hydroxylation and methoxylation of aromatic rings. Ferric reducing antioxidant power assay was used to evaluate this property. 2,3-Dihydroxybenzoic acid was found to be the strongest antioxidant, while mono hydroxylated and methoxylated structures had the lowest activities. A comprehensive structure-activity investigation with density functional theory methods elucidated the influence of compounds topology, resonance stabilization, and intramolecular hydrogen bonding on the exhibited activity. The key factor was found to be a presence of two or more hydroxyl groups being located in ortho or para position to each other. Finally, the quantitative structure-activity relationship approach was used to build a multiple linear regression model describing the dependence of antioxidant activity on structure of compounds, using features exclusively related to their topology. Coefficients of determination for training set and for the test set equaled 0.9918 and 0.9993 respectively, and Q2 value for leave-one-out was 0.9716. In addition, the presented model was used to predict activities of phenolic acids that haven't been tested here experimentally.
Fluorescence quantum yields of matrices used in ultraviolet matrix-assisted laser desorption/ionization.[Pubmed:32469439]
Rapid Commun Mass Spectrom. 2020 Sep 30;34(18):e8846.
RATIONALE: Ultraviolet matrix-assisted laser desorption/ionization (MALDI) is among the most popular soft ionization methods in mass spectrometry. Several theoretical models have been proposed to explain the primary ion generation in MALDI. These models require knowledge of various matrix molecular parameters for simulation. One such parameter is the fluorescence quantum yield. However, the fluorescence quantum yield reported in previous studies remains controversial. METHODS: In this study, we used a commercial and a homemade integrating sphere to measure the absorption and fluorescence quantum yields of several commonly used matrices, including 2,3-Dihydroxybenzoic acid, 2,4-dihydroxybenzoic acid (2,4-DHB), 2,5-dihydroxybenzoic acid (2,5-DHB), 2,6-dihydroxybenzoic acid, 3,4-dihydroxybenzoic acid, 3,5-dihydroxybenzoic acid, alpha-cyano-4-hydroxycinnamic acid, 2,4,6-trihydroxyacetophenone, and ferulic acid. RESULTS: The fluorescence quantum yields of these matrices were determined to be low (<0.08) at low laser fluences and decreased as the laser fluence increased. The fluorescence quantum yields at the typical laser fluence for MALDI are below 0.04 (2,4-DHB and 2,5-DHB) and 0.01 (the other matrices). Shot-to-shot fluctuations of fluorescence intensity and absorption are not directly related to the fluctuation of ions. Possible mechanisms for the decrease in the fluorescence quantum yield as the laser fluence increased were discussed. CONCLUSIONS: The fluorescence quantum yields of these commonly used matrices are much lower than those reported in previous studies. Although fluorescence quantum yield is an important parameter and it is crucial to obtain an accurate value for theoretical models in simulations, the use of fluorescence quantum yield alone is not a sufficient parameter to justify these models.
2,3-Dihydroxybenzoic Acid Decarboxylase from Fusarium oxysporum: Crystal Structures and Substrate Recognition Mechanism.[Pubmed:32421914]
Chembiochem. 2020 Oct 15;21(20):2950-2956.
A 2,3-Dihydroxybenzoic acid decarboxylase from Fusarium oxysporum (2,3-DHBD_Fo) has a relatively high catalytic efficiency for the decarboxylation of 2,3-Dihydroxybenzoic acid (DHBA) and carboxylation of catechol, thus it has a different substrate spectrum from other benzoic acid decarboxylases. We have determined the structures of 2,3-DHBD_Fo in its apo form and complexes with catechol or 2,5-dihydroxybenzoic acid at 1.55, 1.97, and 2.45 A resolution, respectively. The crystal structures of 2,3-DHBD_Fo show that the enzyme exists as a homotetramer, and each active center has a Zn(2+) ion coordinated by E8, H167, D291 and three water molecules. This is different from 2,6-DHBD from Rhizobium sporomusa, in which the Zn(2+) ion is also coordinated with H10. Surprisingly, mutation of A10 of 2,3-DHBD_Fo to His resulted in almost complete loss of the enzyme activity. Enzyme-substrate docking and site-directed mutation studies indicate that residue R233(Delta) interacts with the 3-hydroxy group of 2,3-DHBA, and plays an important role in substrate recognition for this enzyme, thus revealing the molecular basis 2,3-Dihydroxybenzoic acid decarboxylase.
New lignan tyramide, phenolics, megastigmanes, and their glucosides from aerial parts of New Zealand spinach, Tetragonia tetragonoides.[Pubmed:32419958]
Food Sci Biotechnol. 2019 Nov 27;29(5):599-608.
Twenty compounds, including a new lignan amide, were isolated from the aerial parts of New Zealand spinach, Tetragonia tetragonoides (Pall.) Kuntze, which is an edible halophyte. These compounds were identified by mass spectrometry and nuclear magnetic resonance experiments to be N-2,3-dihydroxy-3-(3,4-dihydroxyphenol)tyramine (new compound), methyl 4-hydroxybenzoate, syringaldehyde, ethyl 4-hydroxybenzoate, 3,4-dihydroxybenzoic acid, 2,3-Dihydroxybenzoic acid, coniferyl alcohol, methyl caffeoate, trans- and cis-coumaroyl-beta-d-glucopyranosides, trans- and cis-feruloyl-beta-d-glucopyranosides, caffeic acid, staphylionoside E, canabiside D, apocyanol A, megastima-5,7-diene-3,4,9-triol, 1-O-oleoyl-3-O-beta-d-galactopyranosyl-sn-glycerol, 5,5'-dimethyl-lariciresinol, and kaempferol 3-O-beta-d-glucopyranoside. These compounds were identified in New Zealand spinach for the first time.
Do Aspirin and Flavonoids Prevent Cancer through a Common Mechanism Involving Hydroxybenzoic Acids?-The Metabolite Hypothesis.[Pubmed:32397626]
Molecules. 2020 May 10;25(9). pii: molecules25092243.
Despite decades of research to elucidate the cancer preventive mechanisms of aspirin and flavonoids, a consensus has not been reached on their specific modes of action. This inability to accurately pinpoint the mechanism involved is due to the failure to differentiate the primary targets from its associated downstream responses. This review is written in the context of the recent findings on the potential pathways involved in the prevention of colorectal cancers (CRC) by aspirin and flavonoids. Recent reports have demonstrated that the aspirin metabolites 2,3-Dihydroxybenzoic acid (2,3-DHBA), 2,5-dihydroxybenzoic acid (2,5-DHBA) and the flavonoid metabolites 2,4,6-trihydroxybenzoic acid (2,4,6-THBA), 3,4-dihydroxybenzoic acid (3,4-DHBA) and 3,4,5-trihydroxybenzoic acid (3,4,5-THBA) were effective in inhibiting cancer cell growth in vitro. Limited in vivo studies also provide evidence that some of these hydroxybenzoic acids (HBAs) inhibit tumor growth in animal models. This raises the possibility that a common pathway involving HBAs may be responsible for the observed cancer preventive actions of aspirin and flavonoids. Since substantial amounts of aspirin and flavonoids are left unabsorbed in the intestinal lumen upon oral consumption, they may be subjected to degradation by the host and bacterial enzymes, generating simpler phenolic acids contributing to the prevention of CRC. Interestingly, these HBAs are also abundantly present in fruits and vegetables. Therefore, we suggest that the HBAs produced through microbial degradation of aspirin and flavonoids or those consumed through the diet may be common mediators of CRC prevention.
Precise Probing of Residue Roles by NRPS Code Swapping: Mutation, Enzymatic Characterization, Modeling, and Substrate Promiscuity of Aryl Acid Adenylation Domains.[Pubmed:31894971]
Biochemistry. 2020 Feb 4;59(4):351-363.
Aryl acids are most commonly found in iron-scavenging siderophores but are not limited to them. The nonribosomal peptide synthetase (NRPS) codes of aryl acids remain poorly elucidated relative to those of amino acids. Here, we defined more precisely the role of active-site residues in aryl acid adenylation domains (A-domains) by gradually grafting the NRPS codes used for salicylic acid (Sal) into an archetypal aryl acid A-domain, EntE [specific for the substrate 2,3-Dihydroxybenzoic acid (DHB)]. Enzyme kinetics and modeling studies of these EntE variants demonstrated that the NRPS code residues at positions 236, 240, and 339 collectively regulate the substrate specificity toward DHB and Sal. Furthermore, the EntE variants exhibited the ability to activate the non-native aryl acids 3-hydroxybenzoic acid, 3-aminobenzoic acid, 3-fluorobenzoic acid, and 3-chlorobenzoic acid. These studies enhance our knowledge of the NRPS codes of aryl acids and could be exploited to reprogram aryl acid A-domains for non-native aryl acids.
Effects of Edaravone on Nitric Oxide, Hydroxyl Radicals and Neuronal Nitric Oxide Synthase During Cerebral Ischemia and Reperfusion in Mice.[Pubmed:31882337]
J Stroke Cerebrovasc Dis. 2020 Mar;29(3):104531.
BACKGROUND: The purpose of this study was to investigate the effects of edaravone on nitric oxide (NO) production, hydroxyl radical (OH(-)) metabolism, and neuronal nitric oxide synthase (nNOS) expression during cerebral ischemia and reperfusion. METHODS: Edaravone (3 mg/kg) was administered intravenously to 14 C57BL/6 mice just before reperfusion. Eleven additional mice received saline (controls). NO production and OH(-) metabolism were continuously monitored using bilateral striatal in vivo microdialysis. OH(-) formation was monitored using the salicylate trapping method. Forebrain ischemia was produced in all mice by bilateral occlusion of the common carotid artery for 10 minutes. Levels of NO metabolites, nitrite (NO2(-)) and nitrate (NO3(-)), were determined using the Griess reaction. Brain sections were immunostained with an anti-nNOS antibody and the fractional area density of nNOS-immunoreactive pixels to total pixels determined. RESULTS: Blood pressure and regional cerebral blood flow were not significantly different between the edaravone and control groups. The levels of NO2(-) did not differ significantly between the 2 groups. The level of NO3(-) was significantly higher in the edaravone group compared with the control group after reperfusion. 2,3-Dihydroxybenzoic acid levels were lower in the edaravone group compared with those in the control group after reperfusion. Immunohistochemistry showed nNOS expression in the edaravone group to be significantly lower than that in the control group 96 hours after reperfusion. CONCLUSIONS: These in vivo data indicate that edaravone may have a neuroprotective effect by reducing levels of OH(-) metabolites, increasing NO production and decreasing nNOS expression in brain cells.
Bifunctional Au-Fe3O4 Nanoheterodimers Acting as X-ray Protector in Healthy Cells and as X-ray Enhancer in Tumor Cells.[Pubmed:31613607]
ACS Appl Mater Interfaces. 2019 Oct 30;11(43):39613-39623.
Bifunctional Au-Fe3O4 nanoheterodimers were synthesized by thermally decomposing Fe(III)oleate on gold nanoparticles followed by functionalizing with tiron, 2,3-Dihydroxybenzoic acid, or caffeic acid. These catechol derivatives are antioxidative and thus are predicted to function as superoxide scavengers. In particular, caffeic acid lost its antioxidant capacity, although it was covalently linked through its carboxyl moiety to the Fe3O4 surface. Tiron was shown to bind via its catechol group to the Au-Fe3O4 nanoheterodimers, and 2,3-dihydroxybenzoic was just physisorbed between the oleic acid surface structures. Caffeic-acid stabilized Au-Fe3O4 nanoheterodimers turned out to act as X-ray protector in healthy cells but as X-ray enhancing agents in cancer cells. Furthermore, these functionalized Au-Fe3O4 nanoheterodimers were found to inhibit the migratory capacity of the cancer cells.